
October 25, 2000
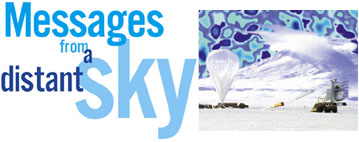
Boomerang
project explores origins, geometry of the universe
By Gilles
Carter '80
At a NASA press conference
on April 26, 2000, an international team of astrophysicists led
by California Institute of Technology professor Andrew Lange '80
presented startling new images of the heavens. To obtain these remarkable
pictures - formed by minute temperature differences in the microwave
spectrum of the embryonic universe - the scientists, who dubbed
their project "Boomerang," built a two-ton telescope armed
with an array of sensitive detectors. Boomerang, which is short
for Balloon Observations of Millimetric Extragalactic Radiation
and Geomagnetics, proved an apt acronym, as the wind-borne instrument,
floated by balloon, made a huge, 10-day loop around the South Pole,
landing as if by magic only 30 miles from the original launch site.
Pictured:
Andrew Lange '80 (right) and Barth Netterfield *95, a member of
the Boomerang team and a professor at the University of Toronto.
Cosmology rarely makes
headlines, but Boomerang's images are big news. The front page of
the New York Times proclaimed that Lange and his team had succeeded
in nothing less than taking clear and detailed pictures of the infant
universe, and that a good measurement of the curvature (or flatness)
of space had been extracted from the data. Boomerang also garnered
an April cover of Nature magazine. "To have such detailed images
is exciting," says cosmologist Wayne Hu '90, an assistant professor
at the University of Chicago, who wrote a technical analysis of
the data for the same issue of Nature. "They're essentially
snapshots of the universe when it was 300,000 years old."
The infant universe?
Look back in time? Measure the curvature of space-time? With data
as clear as Boomerang's, the scientists can smile at the skeptics.
It is remarkable how well the empirical evidence provided by these
images matches up with theory; indeed, Lange says the real credit
should go to the theorists. "It is an incredible triumph to
have predicted the form of the early universe so accurately,"
he says.
Astrophysicists say that
data from Boomerang and other experiments have moved them an Orion-sized
step closer to one of the holy grails of cosmology: establishing
whether the geometry of the universe is curved or flat. Lange explains
that there has been mounting evidence that the matter and energy
with which we are familiar constitute only a small fraction - perhaps
five percent - of the universe. Einstein taught us that all forms
of matter and energy will warp space, creating a lens that can magnify
(or diminish) the image of distant objects. Measuring this effect
is a way to gauge or "weigh" the universe, and to confirm
the presence of the exotic forms of matter and energy that scientists
believe exist.
To investigate the question
of curved or flat, Lange's team, like celestial surveyors plumbing
the sky, had to select a faraway object and determine whether the
light emanating from the object reached Earth on a straight path
or a curved one. The task was to find a suitable distant object,
figure out its size, and determine how big it appeared as viewed
through the lens of space-time.
The object selected was
not a solitary star or galaxy. Instead, they used the tiny fluctuations
in density in the early universe that gravity, acting over billions
of years, would eventually turn into the vast superclusters of galaxies
that we see in the universe today. Lange says, "These embryonic
'seeds' were so vast that they appear today to be roughly twice
the angular diameter of the full moon, despite their great distance."
The difficulty in detecting them, then, is not their size, but the
fact that they are evident only as minute temperature variations,
10 to 100 parts per million of a chilly 2.7 degrees above absolute
zero, and are detectable only at microwave frequencies.
As to looking back in
time, Lange explains that observing these distant objects is a neat
trick of mathematics. Sunlight takes approximately eight minutes
to reach us. When we observe a sunspot flare up, it actually occurred
eight minutes ago. Light from Andromeda, the closest galaxy, takes
over two million years to travel to earth. Beyond the stars and
galaxies, billions of years away, we look back to a time when the
universe was still aglow with radiation. This glow is the cosmic
microwave background, known in the trade as the CMB.
Pictured:
Boomerang's Rorschach-like images are actually high-resolution pictures
of the cosmic microwave background. The black dot in the lower right
is the moon, shown for scale.
Boomerang's splotchy
images are actually high-resolution pictures of the CMB. Lange's
pictures of the primordial universe are thus pointillist compositions
of temperature measurement, but the effect is hardly as serene as
a Seurat. The color-enhanced images look as though a thousand multicolored
bugs simultaneously splattered on the windshield of a speeding car.
When we look at Lange's snapshots, we are as close as we are ever
likely to get to both temporal and spatial infinity. The pictures
represent the ultimate limit of our vision.
Thirty-five years ago,
the Princeton Gravity Group, including professors Robert Dicke,
Jim Peebles, and David Wilkinson, were already building an experiment
to detect the distant CMB. For reasons that are obvious today, telephone
companies were also starting to show interest in microwaves. At
Bell Labs, about 20 miles from Princeton, scientists Arno Penzias
and Robert Wilson pointed a microwave receiver skyward and discovered
a small but consistent reading throughout the universe. At first,
the pair feared there was a problem with the instrument. Frustrated
by numerous attempts to eliminate the extra static, and increasingly
unsure of what they had found, they phoned Dicke. Wilkinson, who
was in the room at the time, remembers the call. "We overheard
certain phrases that made us pay attention. Then Dicke hung up,
turned to us, and said, 'Boys, we've been scooped.' "
Dicke, Peebles, and Wilkinson
had the answer Penzias and Wilson were looking for: The signal was
relic radiation, which first appeared when the universe cooled down
enough to permit subatomic particles to form atoms. The CMB was
exactly what the Princeton scientists had hoped to find: lingering
evidence of the Big Bang. Thirteen years later Penzias and Wilson
were awarded the Nobel prize.
Wilkinson recalls that
when research on the CMB began at Princeton, many in the scientific
community were skeptical. "It took five years for us to convince
everyone that this was really radiation from the Big Bang. We'd
give a talk, and they'd shake their heads; they thought we were
over-interpreting the data. They didn't like the idea of an evolutionary
universe and preferred steady-state theory." Cosmologists were
still inclined to believe that the microwaves came entirely from
atmospheric interference or some other unexplained source.
Princeton was at the
forefront of attempts to remove any lingering doubt by improving
the quality of the CMB signal, including the first aerial observation.
Since water vapor absorbs microwaves, the best signals are obtained
higher and dryer. For this reason, Paul Henry *71, now of AT&T,
designed and flew the first balloon-borne radiometer as part of
his Princeton graduate thesis.
Though the first instruments
used by Penzias, Wilson, Wilkinson, and Henry showed the CMB to
be smoothly distributed throughout the sky, astrophysicists believed
there should be detectable, if tiny, variations in the temperature
of the CMB from one part of the sky to another. The first hint of
these variations came from the COBE (Cosmic Background Explorer)
satellite, launched in 1991.
Lange says, "Theorists
felt that the CMB should not be perfectly smooth. The structures
that we see around us today - stars, galaxies, clusters, and super
clusters of galaxies - were presumably created by gravitational
collapse around ever-so-slightly over-dense regions in the early
universe. These density variations, or ripples, in the early universe
would leave their imprint on the CMB - as we observe it today."
Pictured:
The Boomerang gondola hangs from the long-duration balloon and repeatedly
scans the observing field.
Physicists predicted
that the CMB would, with precise measurement, yield a ghostly impression
- a sort of sonogram of the early universe. If they were right,
and if the ripples in this sonogram could be detected, then the
characteristic angular size of the ripples would, if compared with
a theoretical calculation
of how big they ought to be, provide the lens curvature
measurement which would indicate the geometry of the cosmos.
Boomerang proved they
were right, achieving just that type of image through its precise
measurements. The telescope detected temperature variations of 100
millionths of a degree (0.0001 C). Each of the 16 detectors on board
made 54 million separate measurements during the 10-day flight.
Every one of these 864,000,000 measurements helped form the mottled
Boomerang images, which cover about 3 percent of the sky. "They
made a fantastic high-precision measurement," says Princeton
professor Lyman Page. "The images are wonderful and visually
stunning."
Boomerang measured these
temperature splotches in the CMB at four different frequencies and
produced an extremely clear "power spectrum," showing
how the size of the fluctuations vary with angle, essentially sorting
the CMB into big structures and small structures, just as the graphic
equalizer of a stereo sorts the music into bass and treble. The
results provide striking evidence that the geometry of the universe
is flat.
Lange emphasizes that
Boomerang's contribution is but a small part of a long and complex
quest. He points to several similar projects, which all seem to
have a remarkable number of Princeton faculty and alumni on board.
This, he says, can be attributed to the inspiration found on campus
at Jadwin Hall. "I was lucky enough to have both Dave Wilkinson
and Jim Peebles for freshman physics," Lange says. "I
was one of those goslings that imprints as they come out of the
shell; from the moment I met them, I knew exactly what I wanted
to do."
Wayne Hu agrees. "Professors
Dave Wilkinson and Jim Peebles have trained nearly everyone in the
field; much of the seminal work was done at Princeton."
Indeed, while there has
been much fanfare for Boomerang, Lange suggests that Princeton,
and especially Lyman Page, deserves more attention for an experiment
that preceded Boomerang and showed the first real detail in the
power spectrum of the early universe. "Lyman Page was really
the first to show the universe was flat," Lange says. "Lyman
was the first to discover this characteristic angular scale in the
CMB, which resembles the characteristic note in a musical scale,
that cosmologists now refer to as 'the first peak.'"
One of the next objectives
is to find the harmonics, or overtones, of "the first peak."
Just as it is the overtones that allow our ear to distinguish between
the same basic tone played by a violin or a trumpet, the subsidiary
peaks will undoubtedly reveal far more about the universe. Scientists
were hoping Boomerang would show these subsidiary peaks. As yet,
the lesser peaks have not emerged from the data.
If all goes well, Lange
and other cosmologists expect those subsidiary peaks will be confirmed
by a new satellite, called MAP, designed and built primarily at
Princeton and the Goddard Space Center. MAP promises to chart the
entire CMB in great detail. The satellite will be the sole payload
on a Delta rocket launch at Cape Kennedy next May.
"Cosmology is fast
moving from theology to science," says Page. These experiments
are proving that we live in an evolutionary universe, which long
ago was hotter and denser and is now moving outward at a rapid rate.
This supports the Big Bang theory. We also now know the geometry
of the universe to be flat. "What is truly interesting about
Boomerang," says Page, "is that we've started to put constraints
on the contents of the universe, helping us, for example, to determine
the total number of baryons [normal matter]." As suspected,
and as Boomerang and other experiments seem to confirm, only five
percent of the total mass and energy of the universe is the matter
we recognize - electrons (leptons), and protons and neutrons (baryons).
It follows, of course,
that we know virtually nothing about the 95 percent which constitutes
the rest. Cosmologists refer to the unknown portions as "dark
energy" and "dark matter." It may be only a matter
of time before we determine the nature of the dark matter and dark
energy that fill the universe. One day we may even be able to say
what lies past the periphery, on the other side of the CMB. There
can be no doubt, however, that the last three decades of CMB experiments,
in which Princeton scientists have played such a large part, have
transformed the field and what little we do know about the universe.
Gilles Carter '80 develops
and writes commercial, educational, and documentary
programming for Visionsmith Television.
On the Web
Boomerang project: www.physics.ucsb.edu/~boomerang
|